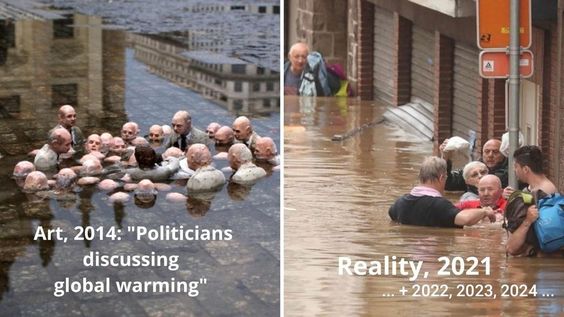
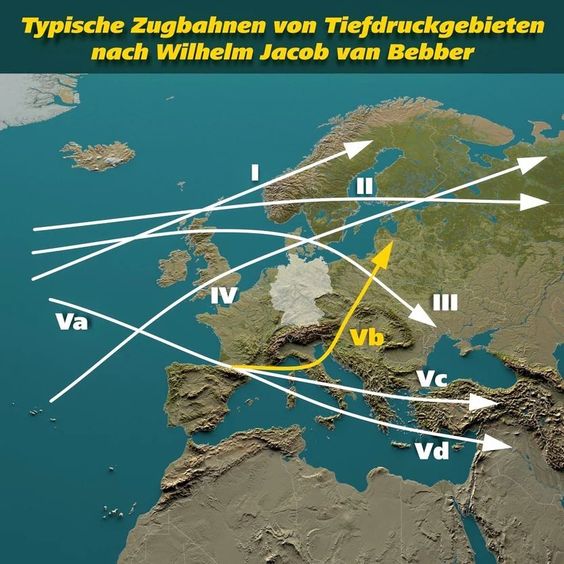
A Vb-type depression will cause very severe weather in central Europe
@MystereClimat – What impact is expected from climate change on these depressions which are developing over the western Mediterranean and moving towards the north-east and which constitute a major natural risk?
According to Volosciuk et al., rising Mediterranean sea surface temperatures amplify extreme summer precipitation in central Europe associated with Vb-type depressions. An experiment by Messmer et al. thus shows that an increase of +5°C in Mediterranean SSTs leads to an average increase of 24% in precipitation over central Europe linked to Vb depressions.
The physical mechanism responsible is the increase in evaporation and atmospheric moisture content over the Mediterranean Sea. Excess precipitable water is transported from the Mediterranean Sea to Central Europe, causing higher extreme precipitation events.
Stefan Rahmstorf, Potsdam Institute for Climate Impact Research, Potsdam, Germany
With current policies the Earth is on track to a warming of around 3 °C above preindustrial temperatures,
a level of heat our planet has not seen for millions of years. Ecosystems, human society and infrastructure are not adapted to these temperatures. Due to non-linear effects, the impacts will be much more severe than just three times as bad as after 1 °C of warming. Land areas will continue to warm much more than the global average, many regions twice as much or even more. Extreme heat will become far more frequent and a major cause of human mortality, making large parts of the tropical land area essentially too hot to live. In addition, extreme rainfall and flooding, droughts, wildfires and harvest failures will increase in frequency and severity. The destructive power of tropical cyclones will also increase. Sea-level rise will accelerate further, and the destabilization of ice sheets will commit our descendants to loss of coastal cities and island nations. The risk of crossing devastating and irreversible tipping points of climate and biosphere will rise to a high level. This 3-degree world is not an inevitable fate, but action to prevent it must be swift and decisive.
What does 3 °C of global warming mean for us? So far, according to the Intergovernmental Panel on Climate Change (IPCC, 2021), we have reached 1.1 degrees of warming, relative to the late nineteenth century (which is generally used as the baseline in this article because it is also the baseline for the Paris target of 1.5 degrees). We are already seeing many negative effects. Three degrees of warming would be nearly 3 times more. However, the effects would be considerably worse than just 3 times the current impacts, as we will see in this chapter.
A useful perspective on a warming of 3 degrees is provided by the Earth’s history. According to current knowledge, one must go back about three million years, to the Pliocene, to find a similarly high global temperature. This already indicates that large parts of today’s biosphere are not adapted by evolution to such a warm Earth. Many species would not survive it. In the Pliocene, our ancestors, the australopitheci, still lived partly on trees.
The course of global temperature over the last 20,000 years since the peak of the last ice age can now be reconstructed quite accurately thanks to numerous sediment and ice cores (Fig. 1). The graph shows three important things:
- 1.Today’s temperatures already exceed the range prevailing in the Holocene and thus the entire history of human civilisation since humans developed agriculture and became sedentary.
- 2.Modern global warming is about ten times faster than the natural warming from the Ice Age into the Holocene, making adaptation massively more difficult.
- 3.Modern warming will last for tens of thousands of years—unless gigantic amounts of carbon dioxide can be actively removed from the atmosphere.
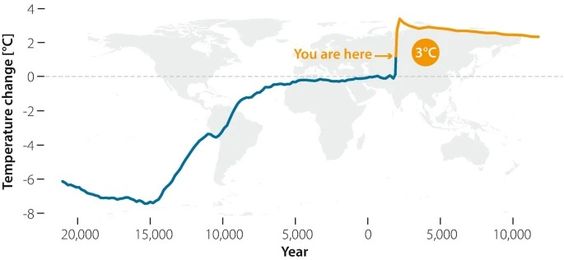
Model simulations at the Potsdam Institute for Climate Impact Research (PIK), which correctly reproduce the ice age cycles of the last three million years (driven by the known Milankovitch cycles of the Earth’s orbit), show that we have probably already added enough CO2 to the atmosphere to prevent the next ice age, which would otherwise be due in 50,000 years. If we heat up the Earth by as much as 3 °C, the natural ice age cycles of the next half million years will probably not occur. A few generations of humans are changing our planet Earth massively and for long geological periods.
The US has already reached around 1.7 °C of warming (Fig. 2). Because the US is a land area, this is not surprising, since many land areas are warming about twice as fast as the global mean, 70% of which draws on ocean temperatures. The average warming of all land areas in 2020 was 2.0 °C. With 3 degrees of global warming, we can therefore expect around 6 degrees of warming on land.
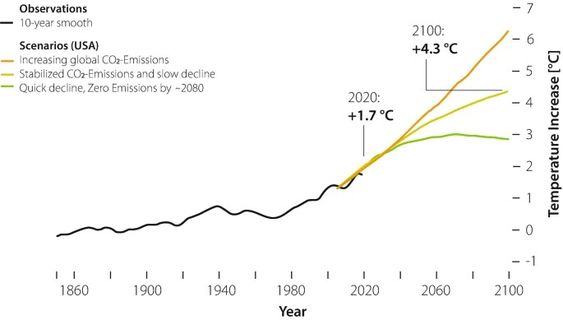
A 6-degree rise in the annual average temperature—that’s a lot. This would make New York roughly as warm as Los Angeles is today. And while some might dream of California weather conditions, this completely new climate will not please farmers or the local flora and fauna at all. It is likely to lead to widespread drought problems, wild fires and forest dieback.
Extreme Heat
Even more important than the average temperatures are the extremes. Where New Yorkers used to groan under a heat wave of 40 °C, it will be an unbearable 46 degrees. Or even more with drying out of soils, which can intensify the heat.
in Europe, the summer of 2003 was called “summer of the century” and claimed around 70,000 heat-related deaths (Robine et al., 2008, pp. 171–175). The peak in excess mortality in France (where the center of the heat was) was significantly higher than the spikes during the Covid 19 pandemic. The city of Paris had to set up refrigerated tents for the many dead in August 2003 because the morgues were overflowing.
For summer 2022 the heat-related mortality in Europe has been estimated as exceeding 61,000.
The human body’s cooling system works by sweating, i.e., by evaporating water on skin surface. How well this works depends on temperature and humidity: the more humid the air, the lower its ability to absorb additional water vapor. The relevant measure of heat stress is the theoretical limit of cooling: the lowest temperature that can be reached by direct evaporative cooling. It is also called wet bulb temperature because it can be measured with a ventilated thermometer wrapped in wet cloth.
According to new research, the human body’s stress limit is at a sustained wet bulb temperature of 35 °C, but even temperatures below 30 degrees can be dangerous, because we must keep our body temperature at around 37 degrees and must be able to dissipate the heat generated by our metabolism and physical exertion. In the heat wave of 2003, wet bulb temperatures of 28 °C occurred in Europe.
At a humidity of 70%, the wet bulb temperature of 35 degrees, which is lethal after a few hours even for healthy people, is reached at an air temperature of 40 °C. Today, this wet bulb temperature is exceeded, anywhere on Earth, only rarely and for brief periods, mainly in the Persian Gulf or on the Mexican coast. According to a recent study (Raymond et al., 2020), the frequency of dangerous values has already more than doubled since 1979 and, in the Persian Gulf, monthly values of sea water temperatures exceeded the 35-degree limit for the first time in 2017—the moisture-saturated breeze from the sea can be deadly at such temperatures. In Qatar, since May 2021, workers are no longer allowed to work outdoors between 10 am and 3.30 pm during summer.
With a global warming of 3 degrees—which, as I said, is equivalent to 6 or more degrees Celsius on many land areas—the areas that are deadly hot during heat waves will expand massively, making it increasingly dangerous to stay outdoors and thus impairing work in agriculture or on building sites, for example.
Extreme Precipitation and Droughts
Temperatures still behave approximately linearly—that is, they increase in proportion to our cumulative emissions of carbon dioxide. Unfortunately, this is not true for many effects of warming. Many physical effects increase more than proportionally. This is true, for example, of the atmosphere’s ability to hold water vapor, which increases exponentially with temperature. This is captured in the Clausius-Clapeyron equation, a basic law of physics describing the saturation vapor pressure of water vapor that has been known since the nineteenth century.
The same increase also applies to the vapor deficit of the atmosphere. This vapor deficit is the amount of water vapor that the air can still absorb at a given relative humidity. This is relevant because as the earth heats, the relative humidity remains approximately constant, and the vapor deficit therefore increases exponentially. It is this vapor deficit of the air that causes soils and vegetation to dry out on hot days, withering crops and increasing the risk of forest fires.
The data show that extreme precipitation has already significantly increased—as climate models have predicted for three decades. This applies to the Earth overall and has now been shown also for many regions (IPCC, 2021). Because of the stronger natural fluctuations on a regional scale and the smaller number of cases of extremes, the signal becomes statistically detectable later for smaller regions. The current report of the Intergovernmental Panel on Climate Change also includes Central and Northern Europe among the regions where an increase can already be detected. In 2020, a study by ETH Zurich also showed a statistically significant increase in extreme rainfall events is observed in these countries (Zeder & Fischer, 2020).
Overall, precipitation increases worldwide with warming because the evaporation rate from the oceans increases by about 2–3% per degree.
Almost the entire increase falls from the sky in heavy rainfall events, for which the amount of water vapor in saturated air masses is important, which, according to the Clausius-Clapeyron equation mentioned above, increases by 7% per degree Celsius of warming—i.e., faster than the water supply through evaporation. As a result, heavy rainfall increases, days with little precipitation become rarer, and periods without precipitation become longer. Overall, therefore, both heavy rainfall events and periods of drought increase.
The destruction that extreme precipitation can cause has been demonstrated by a devastating series of flooding events in 2023. In September 2023 alone, massive floods occurred in Libya, Greece, Spain and in Hong Kong and New York City (Ombadi, 2023).
There are also large regional and seasonal differences in precipitation. Certain regions such as the Mediterranean, the Midwest of the USA, South Africa and Australia are increasingly drying out. What matters for agriculture and natural ecosystems, is agricultural drought, i.e., loss of soil moisture and drying out of vegetation. Drought understood in this way increases even if precipitation remains unchanged, because in a warmer climate water loss through evaporation increases. The current IPCC report also reports an increase in drought caused by anthropogenic warming for the majority of the world’s land areas.
In addition to the simple fact that warm air can absorb more water vapor, there are also changes in atmospheric dynamics. Current research indicates that the persistence, i.e., duration, of certain weather conditions has increased in large parts of Europe in recent decades (Hoffmann et al., 2021). Thus, a few hot days turn into a health-threatening heat wave, or a dry phase into a prolonged drought. This increasing persistence is attributed to a slowing of the general westerly wind circulation, including the jet stream in summer, which is probably related to the strong warming of the Arctic land areas (Coumou et al., 2015). According to a recent study (Voossen, 2021), the Arctic has actually warmed four times as much as the rest of the globe over the last 40 years which flattens the temperature gradient from the tropics to the Arctic which drives the mid-latitude westerly winds. In addition, there are occasional large and persistent variations in the jet stream which reach around the entire northern hemisphere, causing simultaneous extremes there (Kornhuber et al., 2017). A nightmare scenario for some climate researchers is a drought with crop failures simultaneously hitting the large granaries of the northern hemisphere: Western North America, Russia, Western Europe, and Ukraine (Kornhuber et al., 2019).
Already in the drought and fire disaster in the summer of 2010, Russia stopped exporting grain because of crop failures, which led to massive price increases for North African buyers and thereby contributed to the “Arab Spring”, which was partly ignited by high bread prices. Similarly, the revolt in Syria, which began in March 2011, followed the worst drought there in more than a century of weather records (Kelley et al., 2015). Conflict-ridden, weak states can be destabilised by extreme events and crop failures, with implications for global politics.
Tropical Cyclones
Tropical cyclones are a significant hazard in the tropical and subtropical regions of the world. For example, in September 2017, Category 5 Hurricane Maria destroyed large parts of the island of Puerto Rico and caused the loss of more than 3000 lives.
Global warming invests tropical cyclones with additional energy—because these storms draw their destructive power from the heat energy stored in the upper ocean. This is why they only form in regions with water temperatures above 26.5 °C; in more temperate latitudes, the seawater has heretofore been too cold. Climate researchers have therefore been predicting for decades that tropical storms would become stronger. For a long time, however, an increase could not be demonstrated from the observational data. Not because the data did not show an increase (they did), but because it was unclear how reliably the older data captured the strength of tropical storms and whether some tropical storms far from land areas might have been missed before the satellite era.
More recently, a real climatic increase in tropical storm intensity can be seen from the data (Kossin et al., 2020). The current IPCC report states for the first time that the proportion of especially strong tropical storms (categories 3–5) has increased, for which anthropogenic climate change is the main cause. Anyone familiar with earlier IPCC reports’ extremely cautious and restrained statements on this question will understand the significance of this conclusion. In addition, there is evidence that tropical storms can intensify more rapidly, travel more slowly (affecting areas below the storm for longer) and move to higher latitudes—in Europe, for example, approaching the coast of Portugal.
It has long been undisputed that extreme precipitation, which is often the main reason for the devastation caused by tropical storms, has increased due to warming, which again is due to the Clausius-Clapeyron equation and, more particularly, to the increase in evaporation of warmer seawater under the storm.
Hurricane Harvey hit Houston in August 2017 and became the costliest tropical storm in US history ($125 billion in damage), on a par with Hurricane Katrina in New Orleans in 2005. Harvey brought the heaviest rainfall ever recorded in the USA: 1539 mm of rainfall in 4 days at its peak. For comparison: the 3-day precipitation total in the German Ahr valley was 115 mm during the flood disaster in July 2021.
It is also indisputable that rising sea levels due to the warming of Earth aggravate storm surges caused by tropical or other storms. It is often the last additional decimeters that cause the greatest damage, when water reaches areas where no one had previously expected a storm surge. Like Hurricane Sandy in 2012, whose storm surge flooded tunnels of the New York subway. Or Typhoon Haiyan, whose storm surge in 2013 leveled the city of Tacloban in the Philippines and claimed over 6300 lives.
Sea Level and Ice Sheets
The perspective of Earth’s history also helps when it comes to sea level. In the Pliocene, three million years ago, sea levels were between 5 and 25 m higher than today because there was much less ice on the continents. Conversely, at the peak of the last ice age 20,000 years ago, sea levels were 120 m lower than today. The current continental ice masses, especially in Antarctica and Greenland, are so large that they can provide enough water for 65 m of global sea level rise.
Our Australopitheci ancestors in the Pliocene are unlikely to have been bothered by the higher sea level. But our planet’s current coastlines are home to more than 130 cities larger than a million inhabitants, plus other infrastructure such as ports, airports, and some 200 nuclear power plants with seawater cooling (such as Sizewell B on the British North Sea coast). Even 1 m of sea rise would be a disaster. So far, the rise since the late nineteenth century has been around 20 cm, which is already causing problems on some coasts—and this not merely during storm surges. Even normal tidal cycles occasionally cause streets in cities on the U.S. East Coast to be inundated in what is called “nuisance flooding”—not a disaster, but a growing nuisance.
In the case of sea level rise, it appears thus far that the speed of the rise is increasing roughly in proportion to the temperature rise. This means that, with 3 degrees of warming, sea levels should rise roughly three times faster than today. This is partly because the warmer it gets, the faster the continental ice masses melt. The rise in sea level is already accelerating—this is visible not merely in the long data series of harbor tide gauges, but meanwhile even in the satellite measurements that have been available only since 1993 (Fig. 3).
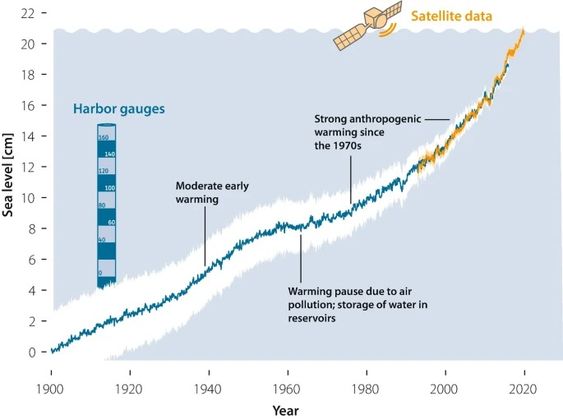
However, there are also more complex effects here that add to this simple logic. Ice does not merely melt on the surface, it can also slide into the sea, or more precisely: it flows like a viscous slow river. If meltwater gets under the ice, ground friction is reduced and the ice flows faster, thus accelerating sea level rise. In Antarctica, moreover, floating ice shelves located in front of the outlet glaciers are gradually disappearing because they are melted from below by warmer sea water. These ice shelves also impede the flow of continental ice, which will therefore accelerate once these shelves disappear.
And it gets even more complicated: continental ice sheets have tipping points. A tipping point is a point at which further development into a fundamentally different state becomes an unstoppable self-sustained process, driven by reinforcing feedback effects. Greenland’s ice sheet has such a tipping point, after which it will melt completely. The reinforcing feedback consists in the fact that, as the approximately 3000-m-thick ice sheet melts from the top, its surface reaches ever lower altitudes and thus warmer layers of air. After a certain point, the ice is destined to melt completely, even without further global warming. The end result is that global sea levels will rise by 7 m due to the loss of Greenland ice. This tipping point is probably somewhere between 1 and 3 degrees of global warming (Robinson et al., 2012).
The situation of the West Antarctic Ice Sheet is similar. Here we are talking about another 3 m of sea leave rise, which is due to a different feedback effect: marine ice sheet instability, which can cause continental ice to slide unstoppably. There are studies suggesting that this tipping point has already been passed so that the loss of this ice sheet has already been triggered (Joughin et al., 2014).
The current IPCC report expects a sea level rise of 70 cm (compared to the late nineteenth century) before the end of this century with a warming of 3 °C. According to the report, the 1-m mark will be reached between 2100 and 2150. However, there are considerable one-sided risks to sea-level rise—that is, it could get much worse if, especially in the Antarctic, large ice masses are destabilized. The IPCC writes that if emissions are high, more than 2 m by 2100 and even 5 m by 2150 cannot be ruled out—a global catastrophe of unimaginable proportions.
This risk assessment is new to the IPCC. In its fourth report of 2007, it had still anticipated a 1990–2100 sea level rise in the range of 26–59 cm for the highest emission scenario (envisioning up to 5.2 degrees of warming), which corresponds to about 41–74 cm relative to the late nineteenth century. In that report, the IPCC remarked that ice sliding might possibly add another 10–20 cm, thus expressing assurance that, even with extreme warming, the sea level rise by 2100 would remain below 1 m.
Several colleagues, myself included, believed at the time that the IPCC was significantly underestimating sea level risks—not least because the measured rise to date was already some 50% faster than in the IPCC’s model scenarios. In addition, the IPCC assumed that Antarctica would contribute practically nothing to the future rise, again in contrast to the ice loss already shown by satellite data. However, anyone in climate research who takes a more pessimistic view of things than the traditionally very cautious IPCC must deal with being accused of “alarmism” in some media—even if their assessment is correct and later shared by the IPCC.
The current IPCC report further warns that sea levels will continue to rise for millennia after global temperatures will have stabilized and that this rise is—with “very high confidence”—irreversible in the foreseeable future. In its landmark ruling on climate protection in 2021, the German Federal Constitutional Court emphasized intergenerational justice. When sea levels rise, countless generations after us will have to suffer the effects of our decisions today.
What is at stake here is not merely the sea level rise in this century, which we may be able to adapt to. Rather, sea levels will continue to rise for millennia, at 3 degrees of warming by about a meter per century, eroding the Earth’s coastal zones, washing away beaches, threatening all infrastructure with ever-increasing storm surge risks, and making permanent coastal cities as we know them today almost impossible to sustain.
The Tipping Points of the Climate System
Regarding ice sheets, we have already mentioned two tipping points of the climate system at which further development becomes an unstoppable self-sustained process and thus gets out of control. There are more such tipping points, because ultimately all that is needed is a reinforcing feedback, a simple non-linearity, as occurs in many physical systems. For example, a kayak will right itself if you tilt it a little to one side—it stabilizes itself in a horizontal position and resists attempts to tip it. But only up to a certain point—beyond which it continues to turn by itself and then stabilizes in a new position: upside down. This critical point is literally the tipping point.
Greenland also has two stable equilibria under today’s climatic conditions: with the ice sheet as we know it today and without it. The ice is self-stabilising because once it is there, the surface of the 3000-m-thick layer of ice is at such a high altitude that the air is too cold for it to melt. This is called ice-elevation feedback. If, on the other hand, the ice were gone, Greenland’s surface—close to sea level and hence not cold enough to form a new ice sheet—would remain permanently ice-free. The Greenland ice sheet was formed in a colder climate during one of the earlier ice ages.
Such tipping points exist not only in physics, but also for ecological systems, which are self-stabilizing, but can also “tip over” when a stress limit is exceeded. The human body regulates its own temperature—up to a critical heat limit, above which the self-cooling system is overloaded, organs increasingly fail and the person finally dies. So, we too have our personal tipping point. This also applies to societies—the fall of the Berlin Wall was a tipping point of the GDR state. The term tipping point does not describe a value judgment, but merely a certain type of dynamic; the change that is triggered can of course also be desirable, that is in the eye of the beholder.
An overview of the most important tipping points of the climate system is provided in Fig. 4. All these tipping points are in danger of being exceeded at 3 degrees of global warming. For some, such as the Greenland ice sheet and the West Antarctic ice sheet, this is very likely, and for the Arctic summer sea ice cover and the Earth’s coral reefs it even certain. The IPCC concludes that at 2 degrees of warming, almost all coral reefs will die; if we limit this to 1.5 degrees, we could still save 10–30% of the corals. Our planet has already been in a global coral die-off since 2015 (Hughes et al., 2018).
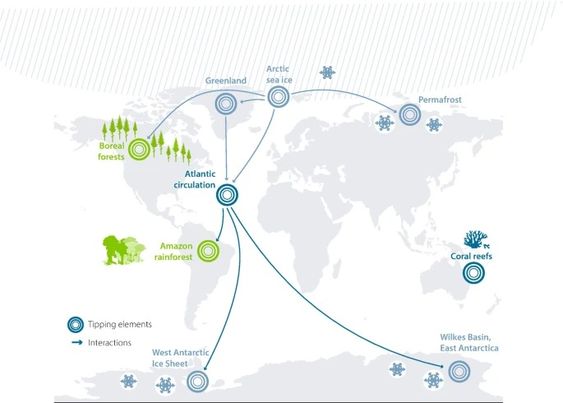
The Atlantic overturning circulation (sometimes called the Gulf Stream system) is a large circulation of the Atlantic Ocean in which warm surface water flows from the South Atlantic across the equator to the far north of the Atlantic, where it cools and releases heat into the air. The whole thing works like a central heating system for the North Atlantic region all the way to Europe. This current is endangered above all by freshwater input due to increased precipitation and ice melt. Freshwater is lighter than salt water and thus impedes the sinking of the water into the depths and thus the driving force of the Atlantic overturning circulation (Rahmstorf, 2024).
Models suggest a weakening of the current due to global warming, but its extent is uncertain, ranging from very small to 50% this century. There is serious evidence that many models systematically overestimate the stability of the Gulf Stream system. A striking cooling of the waters in the subpolar North Atlantic since the middle of the twentieth century indicates a weakening of 15% so far (Caesar et al., 2018). Several studies published since 2021 already found “early warning signs” that we are approaching the tipping point of the Atlantic overturning circulation, possibly even already in the next few decades (e.g. Boers, 2021). If this is confirmed, it will be extremely worrying.
The effects of a breakdown of the flow would be massive and partly unforeseeable, ranging from extreme weather in Europe to the collapse of important ecosystems in the North Atlantic to increased sea level rise on the US coast (up to an additional 1 m).
The rainforests of the Amazon region are already directly affected by climate change. Satellite data and on-site measurements have shown that increasing droughts are transforming the Amazon forest from a carbon sink into a carbon source (Brienen et al., 2015). Already today, parts of the tree populations are not able to cope with the new climate conditions and are dying. At least as important is the expansion of agriculture and the associated deforestation, which exacerbate the effects of climate change. As a result, the Amazon forest is losing resilience as deforestation continues. The tipping point leading to widespread loss of this unique ecosystem will be reached at lower global warming the more deforestation occurs. Today’s forest loss is already estimated at 20%.
With increasing warming, the existence of coniferous forests in the north, which are adapted to cold climatic conditions, may also be increasingly threatened, among other things by fire and insect infestation. In recent years, there have already been extensive forest fires in Canada (e.g., Fort McMurray 2016 and the record-breaking fire season of 2023) and Russia (2010 in the European part) and even within the Arctic Circle (2017 in Greenland, 2018 in Sweden). In the transition zone of the northern forest belt to the steppe, tree stand regeneration may be threatened by increasing drought and heat stress.
A current focus of research is the risk of a cascade of tipping points that trigger one another like dominoes. For example, ice melt in the Arctic Ocean and on Greenland could dilute the North Atlantic water with fresh water to such an extent that the Atlantic overturning circulation would cease. This in turn would shift the tropical rainfall belts and could destabilize parts of the Amazon forest and the monsoons. And as if this were not enough, it could drive the Antarctic ice sheets beyond their tipping point. A quantitative assessment of these risks is still not possible.
The IPCC also attributes a strongly growing importance to tipping points. While the term “tipping point” was mentioned only 27 times in the 5th IPCC report, it was already mentioned 97 times in the 6th report. In its 2023 Synthesis Report of the latter, the IPCC concludes that “risks associated with large-scale singular events or tipping points, such as ice sheet instability or ecosystem loss from tropical forests, transition to high risk between 1.5 °C and 2.5 °C” global warming.
Self-Amplification of Global Warming
There is much public discussion about whether, beyond subsystems, global warming as a whole could, beyond a critical point, become an unstoppable self-amplified process. Mostly, the release of methane from permafrost is mentioned here as a reinforcing feedback. In 2018, a study on this topic appeared in the scientific journal Proceedings of the National Academy of Sciences, which made a big media splash as the “hothouse Earth paper” (Steffen et al., 2018).
The study investigated the extent to which feedback effects in the carbon cycle, which have not yet been taken into account in climate models, could exacerbate global warming. Not only methane release from permafrost was estimated, but also CO2 release from dying or burning forests and a decreasing CO2 uptake by the oceans.
The permafrost region is a globally significant carbon reservoir, which contains 1300–1600 billion tons of carbon and thus probably 50% of the total carbon stored in the soil worldwide. The permafrost areas have already warmed by up to 4 °C between 1990 and 2016. When the permafrost thaws, the soil carbon is decomposed by microbes. This could reduce the carbon stored in the permafrost by 15% by 2100.
Estimates of the carbon stored in living and dead plant material in the Amazon region (above and below ground) range from 80 to 120 billion tons. If this stored carbon were to be completely released in an extreme case, this would correspond to the amount of fossil CO2 emissions that are currently released into the atmosphere in 8–12 years.
The result of the calculations (which unfortunately was somewhat neglected in many media reports) was that a warming of 2 degrees could become a warming of up to 2.5 degrees—if, as mentioned earlier, the carbon cycle changes and feedbacks are triggered.
This is by no means harmless and significantly exacerbates the climate crisis—but it does not mean that a global tipping point towards runaway warming has been passed. Fortunately, this risk is still considered very low, even if it cannot be completely ruled out. The methane problem should in any case be taken seriously, but is probably less dramatic within this century. In the long term, however, it is quite serious because thawing permafrost will create an uncontrollable source of greenhouse gas emissions for many centuries to come, which is likely to lead to further warming even after direct anthropogenic emissions will have been reduced to zero.
Conclusion
Without immediate, decisive climate protection measures, my children currently attending high school could already experience a 3-degree warmer Earth. No one can say exactly what this world would look like—it would be too far outside the entire experience of human history. But almost certainly this earth would be full of horrors for the people who would have to experience it. Weather chaos with deadly heat waves, devastating monster storms, and persistent widespread droughts that could trigger worldwide hunger crises. Rising sea levels that devastate our coasts. Collapsing ecosystems, devastating species extinctions, burning and withering forests, acidified oceans. Failed states, huge numbers of people on the run.
This sounds dark and dystopian and I find it hard to write it while thinking of my children. But it is likely. Most of this was predicted long ago and is now already being observed in its beginnings, which are by no means harmless for those affected. We must soberly face the fact that the conditions in a 3-degree world will most likely not “only” be three times worse than in a 1-degree world, considering the non-linear effects and the tipping points. I am not sure whether the more or less civilized coexistence of humans we enjoy now will endure under such conditions. Personally, I consider a 3-degree world to be an existential threat to human civilization.
What gives hope is that this 3-degree world is not an inevitable fate. It is still possible to limit warming to near the 1.5-degree mark—which was unanimously agreed by all countries in Paris in 2015 and to which almost all politicians in my country pay lip service. Global climate policy is certainly making progress: With the measures announced at the climate summit in Glasgow, the limit of 2 degrees is within reach, if these measures are not only promised but consistently implemented. And the International Energy Agency IEA has presented in September 2003 a feasible pathway to limiting global warming to 1.5 °C, kept open by the phenomenal global growth of renewable energies (International Energy Agency, 2023).
Limiting the temperature to 2 degrees is not enough. In order to meet the 1.5 degree target, the world must finally switch into serious crisis mode, as the young people of Fridays for Future quite rightly demand. Climate protection must be given the highest priority.
Rahmstorf, S. (2024). Climate and Weather at 3 Degrees More. In: Wiegandt, K. (eds) 3 Degrees More. Springer, Cham. https://doi.org/10.1007/978-3-031-58144-1_1
Comments are closed.